 |
Our Techniques
|
In this section we discuss the research
techniques used in our group, and how we use them in tackling scientific
problems.
* Coming soon; segment under construction
Neutron Scattering
The molecular-level mobility and structure of polymers
and proteins occur on timescales of picoseconds - nanoseconds and
length scales of angstroms - nanometers. We use neutron scattering
to investigate these time and spatial scales.
Neutron scattering is similar to X-ray scattering, except neutrons
interact with the nucleus of the atoms, whereas electrons interact
with the electron cloud of the atoms in X-ray scattering. While the
interaction strength between X-rays and atoms depends on the size of
the electron cloud, the interaction strength of a neutron with an
atom varies randomly with atomic number. In addition, neutron scattering
can capture not only the structure, but also dynamics on small length scales.
Dynamics:
We use quasi-elastic neutron scattering [QENS] to measure polymer dynamics.
To cover timescales varying from 1.3ps 2ns, we use two instruments:
the Disk Chopper Spectrometer [DCS] (1.3 - 50 ps, 4 -11 Å) and the
High Flux Backscattering Spectrometer [HFBS] (240 ps - 2 ns, 3.5 - 10 Å).
These measurements are made at NIST Center for Neutron Research
in Gaithersburg, MD.
The instruments measure scattered intensity as a function of
energy and spatial scale. The energy the neutron exchanges with the atoms in the
sample is in the frequency domain, therefore we inverse-Fourier transform the data to
the time domain (see Figure below). Decay in S(q,t) as a function of time indicates
mobility on the timescale of the measurement. We can fit this decay to a stretch
exponential equation, and extract physically relevant parameters such as the
polymer relaxation time and fraction of mobile atoms in the system.
In addition, S(q,t) allows us to compare experimental data with MD simulation data
providing a way to verify MD simulations.
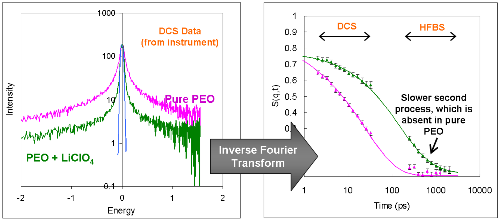
Left: Raw DCS data for pure PEO and PEO + LiClO4
Right: Data from both instruments transformed to the time domain
Structure:
Small-angle neutron scattering [SANS] is a technique we use to measure structure
on length scales of angstroms nanometers. SANS relies on a contrast in neutron
scattering-length densities between the structure to be detected and the surrounding medium.
For example, we have used SANS to investigate the structure of nanoparticle-filled solid
polymer electrolytes (PEO + LiClO4 + Al2O3). The scattering length density of the
alumina nanoparticles is sufficiently different from the surrounding PEO/LiClO4 medium,
and therefore nanoparticle aggregation can be observed. The figure below illustrates
intensity as a function of the wavevector q, (q is inversely proportional to the spatial scale)
for this system. The feature at large q is associated with the primary nanoparticle size,
and the increase in intensity with decreasing q indicates nanoparticle aggregation.
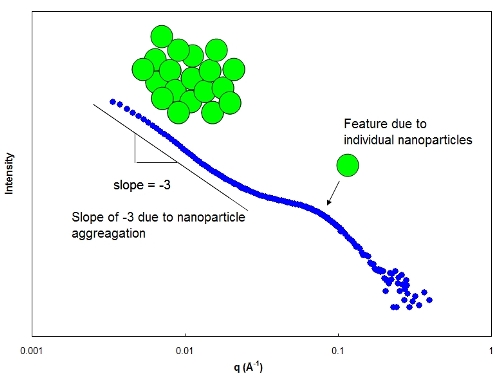
SANS graph depicting nanoparticle aggregation in PEO melt
Go to top
Differential Scanning Calorimetry
We probe the thermal properties of soft matters with Differential Scanning Calorimetry [DSC].
During the cooling and heating scan, when thermal transition occurs, a shift of heat flow supplied to the sample will be observed.
The temperature of the heat flow change can be used to determine the melting, crystallization, and glass transition temperature of the material.
By integrating the total heat flow during a melting and crystallization process, we can estimate the degree of crystallization of a semi-crystalline sample.
The current DSC model we have is TA Instrument Q2000 DSC and the lowest accessible temperature is -90 Celsius..
Go to top
Broadband Dielectric Spectroscopy
We use BDS to measure the ionic conductivity of our solid polymer electrolyte samples.
Conductivity quantifies how fast the ionic species are moving in the polymer host. An example
of conductivity versus temperature data for a solid polymer electrolyte sample is given below.
The sharp decrease in the conductivity at temperatures below 60C occurs because the polymer host
(polyethylene oxide) crystallizes at this temperature.
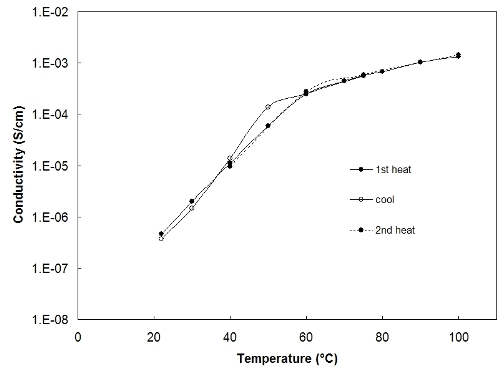
Conductivity measurement using broadband dielectric spectroscopy
Go to top
Molecular Dynamics Simulation
Our group uses molecular dynamics [MD] simulation to study the structure and dynamics on a molecular level. MD simulations solve Newton's
equations of motion using a set of model equations to describe the inter-atomic forces. This technique allows us to record the time-evolution of trajectories of individual
atoms and gain insight into phenomena on a molecular level that is not observable through experiment alone. For example - we can isolate the contributions of individual
atoms to dynamics measurements or look at pair correlations of specific types of atoms. Simulation also allows the visualization of molecular scale phenomena.
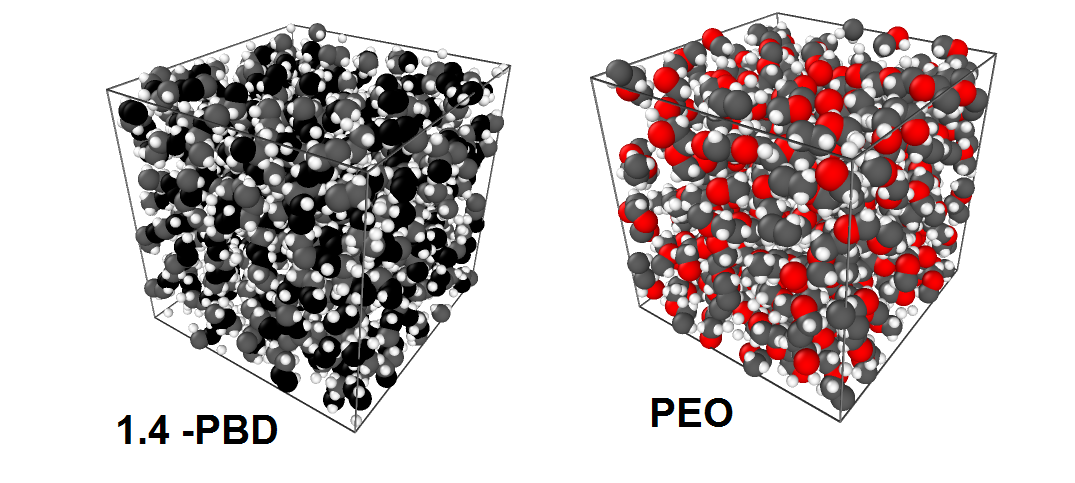
MD atomistic simulations of polymer melts.
Go to top
Coarse-Grained Simulation
A limitation of atomistic molecular dynamic is that the level of detail makes the computational coasts
of driving the simulation expensive for even our most modern computers. Coarse-graining is a simulation
technique that allows us to reduce the detail of a simulation so that we can obtain good statistics on
a particular phenomenon of interest.
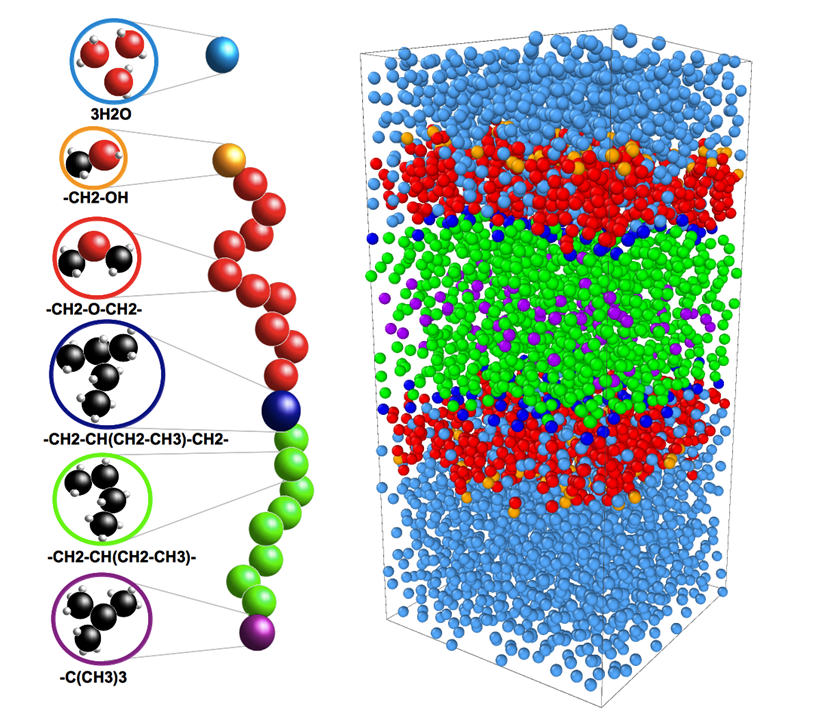
Coarse-grained simulations of PEO-b-PEE copolymer membrane.
Go to top
|