Research Topics
- Electrocatalysis
- Catalysis by doped oxides: M-CeO2 systems
- Heterogeneous Catalysis
- Polymers and Enzymes for Energy Applications
Electrocatalysis
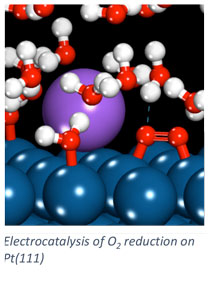
Electrodes in fuel cells and electrolyzers catalyze electrochemical reactions. The design of effective electrocatalysts drives the rates of these reactions and the resulting electrochemical cell efficiency. In our group, we apply computational chemistry techniques to investigate electrocatalytic processes and the electrode/electrolyte interface. Elementary processes are difficult to probe experimentally at the electrode/electrolyte interface, and computational tools help us determine how the catalyst impacts transformations in this interfacial region. Quantum chemistry based methods investigate surface processes including adsorption and surface reaction. Force-field based methods model the longer length and time scales associated with the dynamic electrolyte at the electrode surface.
We apply computational chemistry methods to a number of systems of application emphasis including alkaline and proton exchange membrane fuel cells, direct borohydride fuel cells, oxidation of bio-derived chemicals to value added compounds, and carbon dioxide electrolysis. Specific emphases in our modeling approaches include the consideration of elementary reaction rate constants, specific ion adsorption effects on catalytic processes, and multi-scale modeling of the electrode/electrolyte interface.
We collaborate with a number of experimentalists and theorists in the area of electrocatalysis, including Mike Hickner (Penn State MATSE), John Flake (Louisiana State U.), Susan Sinnott (U. of Florida), Wenzhen Li (Michigan Tech), and Janna Maranas (Penn State CHE).
Catalysis by doped oxides: M-CeO2 systems
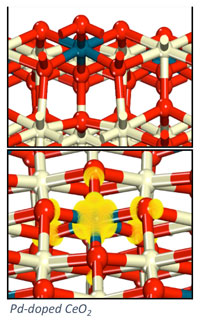
Doped oxides (M1-M2Ox) can offer tunable properties for optimization of catalytic performance. For example, an “active” and expense metal can be doped into the surface of a high surface area support to provide greater oxidation activity, allowing for improved performance and ideal dispersion of the expensive material. Our work on doped oxides has concentrated on the design of metal-doped ceria (M-CeO2) materials for hydrocarbon oxidation and desulfurization. Electronic structure methods, specifically density functional theory, are used to examine surface reaction processes involved in hydrocarbon conversion. Our goal is to predictively design stable and active doped metal oxides for efficient oxidation catalysis.
Our work on doped oxides has relevance to a number of catalytic applications include hydrocarbon reforming for H2 or syngas production, gasifier effluent clean-up, catalytic combustion, and partial oxidation for alkane conversion to higher value chemicals. Our computational work emphasizes use of DFT+U methods and the evaluation of their reliability to predict catalytic properties as well as the integration of reactive force-field methods to examine reactivity in the complex structures that occur under reaction environments.
We collaborate with a number of other faculty in research area of doped metal-oxides, including Kerry Dooley (Louisiana State U.), Matthias Batzill (U. of South Florida), Adri van Duin (Penn State Mech E), and Jason Weaver (U. of Florida).
Heterogeneous Catalysis
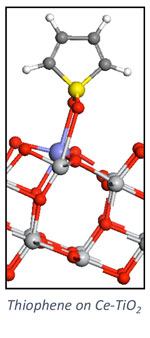
Our group pursues a number of research projects in the area of heterogeneous catalysis. These projects are pursued collaboratively with experimentalists towards the development of catalytic materials and methods for catalyst investigation. Research topics include the development of solid adsorbents for adsorptive desulfurization (collaborative with Chunshan Song, Penn State EME), carbon dioxide hydrogenation (collaborative with Chunshan Song and Xinwen Guo (Dalian U. of Technology, China), and alkylation reactions in zeolites (collaborative with Chunshan Song and Xinwen Guo). We pursue a number of collaborative projects with the group of Robert Rioux (Penn State CHE) include semi-hydrogenation of acetylene and characterization of nanoparticle catalysts.
Polymers and Enzymes for Energy Applications
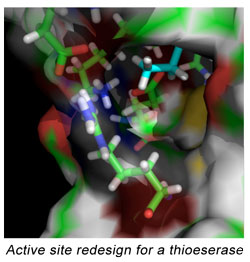
In addition to heterogeneous catalysis, we collaboratively pursue a number of energy conversion processes where our expertise in electronic structure methods can motivate materials design. In collaboration with Scott Milner (Penn State CHE) and Enrique Gomez (Penn State CHE), we are investigating charge transport in polymer materials and across polymer interfaces, of relevance to the development of organic electronics including organic solar cells. Ion transport in ionic polymers is investigated with electronic structure methods in collaboration with Ralph Colby (Penn State MATSE). Through collaboration with Costas Maranas (Penn State CHE), we are integrating quantum chemical methods into a computational framework for enzyme design.