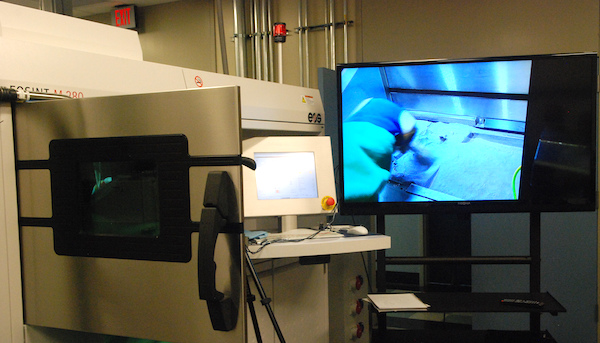
Electronics such as these used in additive manufacturing could look much different in a future with efficient electricity-conducting plastics.
Researchers find potential key to a future of flexible electronics
3/5/2019
UNIVERSITY PARK, Pa. – Conjugated polymers, plastics that conduct electricity like copper or silicon, hold promise for the creation of flexible electronics such as bendable displays and implantable biomedical sensors. Understanding charge transfer in these materials is key to creating such next-generation electronics and is the focus of a three-year, $1.3 million grant from the National Science Foundation (NSF).
The fundamental question this work aims to address is: What governs how quickly electrons move through polymeric materials that make up flexible electronics? This is important because if electrical charge is moving too slowly, then electronics themselves will perform slowly. Conjugated polymers in general exhibit lower charge mobilities than silicon, which is what non-flexible, conventional electronics are made of.
“If electrical charge is moving too slowly, then your display might refresh in minutes as opposed to 60 times a second,” Enrique Gomez, professor of chemical engineering and principal investigator on the NSF grant, said. “Or, your sensor might respond so slowly that it might miss being able to detect something simply because the electronics are not fast enough.”
These charges move along molecular chains, or “backbones.” The chains are on the scale of 10-50 nanometers. So, to cross a macroscopic distance, a molecular charge has to hop to a different chain. But in doing so, the charge slows down. This raises issues in the design of materials for flexible electronics that have design criteria different from regular electronics.
“Our goal is to design conducting materials that perform under whatever needed design criteria,” Enrique said. “So, you need to be able to develop some sort of description of how the molecular parameters, such as the chemical structure or molecular weight, will affect macroscopic properties. Basically, how will that affect mechanical properties and the ability to move charges?”
For example, the design criteria for some displays is to have transistors with charge mobilities of around eight square centimeters per volt-second. Currently, the best polymeric materials can reach about 10 square centimeters per volt-second, which is slightly more, but variability is likely to lead to failure. “If we could design materials so that instead of mobilities of 10 [square centimeters per volt-second], you could reliably reach 20, it would make it more likely that someone could put a product like that into market,” Gomez said.
So, the challenge is to create a flexible conjugated polymer that consistently exhibits high charge mobilities. The researchers demonstrated that a potential solution is nematic order. Nematic order means that the backbones align themselves all in the same direction, much like the strands in a box of uncooked spaghetti. Using an analytic model, the researchers demonstrated nematic order leads to an enhancement in charge mobilities compared to when the chains are pointing in random directions.
Furthermore, as per their analytic model, the researchers predict that there is a quadratic dependence based on the molecular weight of the chain. “We predict that as the molecular weight increases, charge mobilities would increase, and that is a direct consequence of how quickly charges can move through the backbone as opposed to having to hop between the backbones,” Gomez said. “We may be able to predict what molecular weight is needed to achieve fast transport for a given polymer. In other words, we can almost give you molecular weight cutoffs, meaning anything below this molecular weight hurts your ability to move these charges.”
Gomez stresses that the results of their work are rather general.
“We argue that this is a fundamental result, it’s analytical, not a simulation,” he said. “This is an underlying effect that must be true for chain-like semiconductors, and our assumptions are pretty straightforward.”
While there are still questions as to the effect of nematic order on charge transport in conjugated polymers, Gomez notes that this is a significant step in understanding how charges move through these materials. In turn, it is possibly getting closer to the production of a new breed of electronics, from flexible screens to flexible solar cells to implantable biomedical devices.
“This work brings us a step closer to designing new materials for flexible electronics,” Gomez said.
The research team’s findings were published in ACS Central Science, a journal of the American Chemical Society. Other researchers include Scott Milner, William H. Joyce Chair Professor of Chemical Engineering, and Wenlin Zhang, who participated in this research while a Penn State doctoral candidate in chemical engineering and is now a post-doctoral researcher at the University of Michigan.
MEDIA CONTACT:
Jamie Oberdick
jco11@psu.edu